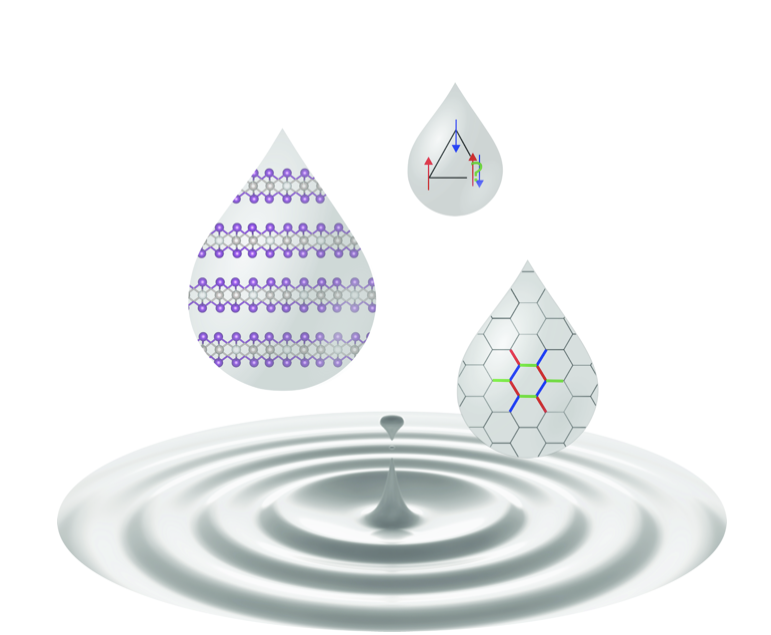
Review written by Mulan Yang (Chemistry, G3) and Brianna Hoff (Chemistry, G3)
One of the main goals of materials science is to develop new materials for fulfilling the various applications we see all around us, from the batteries required to keep our phones running to the plastics we use to store food and drinks. One particular niche is quantum materials science, which focuses on the study and development of materials whose electrons behave differently from how we would expect based on classical models. Quantum materials are especially exciting to study because they have the potential to store information in their electrons more effectively, which is the basis of quantum computing. Finding the perfect material that can be employed in quantum computers would allow tremendous data processing at incredible speeds.
The Cava group at Princeton University is working towards developing such materials and recently published a paper on their synthesis and measurements of the new compound α-RuI3. Due to its unique structure and constituent elements, they proposed that this material could be a Kitaev quantum spin liquid. To fully appreciate the significance of this finding, it is important to first understand what a quantum spin liquid is, and why it is important.
In quantum mechanics, electrons have a property called spin that describes their angular momentum. If we think of an electron as a tiny magnet, spin-up or spin-down means the magnet is positioned with north facing up or down. The way these spins are arranged in a material plays a significant role in determining how it functions. For instance, magnets stick to your fridge because the electron spins are mainly ordered in one direction, whereas a piece of paper would fall because the net alignment of its spins is zero. If we consider an ideal system at absolute zero (0 Kelvin), there is no thermal energy disrupting the electrons. Because of this, all the electrons in the system will spontaneously align themselves into their lowest energy state [1]. Above 0 K, the electrons in a material may have enough energy to vibrate, resulting in an unordered system; the exact temperature and behavior are highly material-dependent.
Under special circumstances, however, there is no single lowest energy state for a system, resulting in a state called a quantum spin liquid (QSL). In the simplest model of this phenomenon, picture three electrons arranged in an equilateral triangle and aligned on one axis, with antiferromagnetic interactions; in this conformation, every electron wants to be the opposite spin of its neighbors. If electron 1 is spin-up and electron 2 is spin-down, the third electron could be spin-up, which would be energetically favorable with respect to electron 2, but this would be unfavorable with respect to electron 1. Likewise, electron 3 could be spin-down to align antiferromagnetically with electron 1, but then it would align unfavorably with electron 2. Thus, the system is ‘frustrated’ because there is no single lowest energy ground state at 0 K. This lack of one preferred ordering pattern means the symmetries remain unbroken, resulting in a QSL [1].
Although scientists have known about QSLs for decades, they were complicated systems to study because they could not be derived exactly from a realistic model. That changed in 2006, when Prof. Alexei Kitaev, at the California Institute of Technology, developed a model of electron spins on a 2-dimensional (2D) honeycomb lattice. This arrangement made it much more mathematically feasible for theorists to study [2]. In this model, imagine a lattice arranged like a honeycomb or tessellated hexagons (see figure for reference), with an electron at each vertex. The spins of the electrons forming each bond have a preferred orientation along an “easy” axis of lowest energy for the overall spin configuration. However, due to the hexagonal lattice, there is no way to arrange the system so that every spin is along its easy axis. Thus, the system fluctuates between many different possible configurations that are all of the same energy.
QSLs can never be practically observed because reaching 0 K is impossible, but finding potential QSL candidates is an important stepping stone in developing materials for quantum computing and data storage. By implementing materials with properties characteristic of QSLs into real-life devices, many applications can be realized, even if the specific properties of the materials are not [3]. While multiple materials have been studied as Kitaev QSL candidates, α-RuCl3 (α denotes a specific structure of RuCl3) is of particular interest as a relatively simple material to make. It adopts a layered honeycomb lattice structure and fulfills the other requirements necessary for a Kitaev QSL [1]. In their recent work, Dr. Danrui Ni and other researchers in the Cava lab prepared a related material, α-RuI3, and proposed it as a candidate Kitaev QSL.
RuI3 can exist in different structures, but until recently, synthesis of the material resulted in a crystal consisting of 1-dimensional (1D) chains, which would not be able to support a Kitaev spin liquid state [4]. However, in 2022, Ni et al. altered the synthesis of RuI3 and utilized high pressure to make a new polymorph, α-RuI3, which crystallizes in stacked honeycomb layers just like in α-RuCl3. The researchers conclude that putting the material under high pressure may have pushed the system towards this type of structure because it is more tightly packed than the 1D chains. Notably, this material is stable for multiple days at normal pressures and in air, enabling its use and characterization without specialized equipment to maintain inert conditions. In fact, α-RuI3 maintains its structure longer than α-RuCl3 does under the same ambient conditions.
To elucidate the structure of α-RuI3 in greater detail, the researchers used single crystal x-ray diffraction, a technique involving shooting a crystal with x-rays and measuring how the path of the x-rays is changed when they bounce off the atoms in the crystal. They found that within each layer, the six I- ions are octahedrally coordinated to a Ru3+ ion. Although each layer looks very similar to those in α-RuCl3, they are separated by approximately 6.3 Å (1 Å = 10-10 m), compared to 5.7 Å in α-RuCl3. Because the layers are further apart, the Ru3+ ions may be less able to communicate with each other and therefore the layers may show weaker magnetic coupling.
A Physical Property Measurement System (PPMS) was used to measure the magnetic and electronic properties of the newly synthesized α-RuI3 phase. This instrument can reach down to temperatures close to absolute zero. Ni et al. confirmed that down to 1.8 K, α-RuI3 displays no evidence of 3D magnetic ordering, as expected of a QSL. Interestingly, when looking at its electronic properties, they found a major difference between α-RuI3 and α-RuCl3. Even at such low temperatures, α-RuI3 behaves like a metal. In contrast, α-RuCl3 is known to be an insulator. They also performed computational simulations to support their experimental findings.
Overall, this research by Ni et al. has worked to expand the library of quantum materials that can be studied. Future research on a material that is both metallic and has Kitaev spin interactions could be interesting, especially to understand why it differs from α-RuCl3. Increasing our understanding of these and similar materials can help scientists find more Kitaev candidates and discover how to use them in applications.
The original article discussed here was published in Advanced Materials on November 28, 2021. Please follow this link to view the full version.
The authors of this review article have also listed some papers for readers interested in exploring this topic further:
[1]: Takagi H. et al. Concept and Realization of Kitaev Quantum Spin Liquids (2019).
[2]: Kitaev A. Anyons in an Exactly Solved Model and Beyond (2006).
[3]: Winter S. M. et al. Models and Materials for Generalized Kitaev Magnetism (2017).