Review written by Cecilia Panfil (CHM, 2022) and Alexandra Libby (PNI, GS)
Abstract
Despite Jupiter’s aurora being the brightest in the solar system, the mechanism of its occurrence is not well understood. One peculiar phenomenon on Jupiter is the large quantities of protons in its magnetosphere. Recently, Dr. Jamey Szalay and his team were able to use data from the Juno spaceship to observe the protons flying away from Jupiter. This provides evidence that the protons are coming from Jupiter itself. The electric fields which drive protons away from Jupiter are likely intimately related to Jupiter’s auroral fields. With their novel observation, Szalay et al. provide a clue towards Jupiter’s complex auroral interactions.
“There was an ongoing mystery on where all the protons surrounding Jupiter came from. Due to Juno’s unique polar orbit and advanced plasma instrumentation, we flew right through and observed intense beams of protons streaming away Jupiter. This is really exciting, because for the first time we have been actually able to experimentally constrain how Jupiter sheds material into its surrounding magnetic bubble." - Jamey Szalay
Jupiter is the largest planet in our solar system. While there are many fascinating processes connected to Jupiter, including its water bearing moons and the over a century long storm that is the giant red dot, here we focus on Jupiter's aurora. As residents of planet Earth, we are familiar with Earth’s aurora—the Northern and Southern Lights—the eerie bluish green lights visible during the winter and summer months, respectively. Auroras occur when charged particles, such as protons or electrons, interact with a planet’s magnetosphere. If a planet has a magnetic field, then ions (i.e., atoms’ missing electrons) and other charged particles will be affected by it; a planet’s magnetosphere is this region of influence. Charged particles are often confined to travel along magnetic field lines, like their own train tracks. Multiple processes can speed these particles up towards the planet, leading them to collide in a burst of light which forms the spectacular blue-green phenomenon.4 The part of the atmosphere containing charged particles is called the ionosphere. On Earth, the aurora is formed as a consequence of the interaction of Earth’s magnetosphere with solar material flowing past Earth, a phenomenon called solar wind. The interaction between charged particles and a planet's magnetic field is strongest at the poles, or where the magnetic fields point perpendicularly out of the planet.
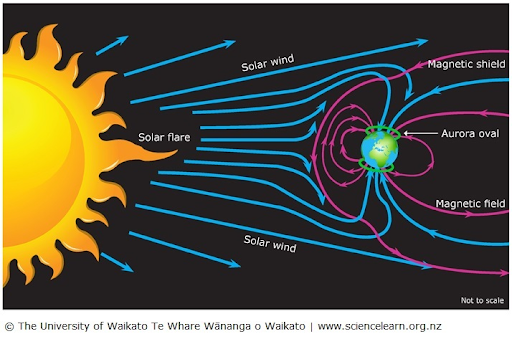
However, it turns out that the precise dynamics of aurora formation on Jupiter, and how they fit the picture we’ve formed from auroral observations on Earth, are not fully understood. There are interesting differences between how the ionosphere interacts with the magnetosphere on the two planets, which sheds light on the various forms auroras can take. Since Jupiter has the brightest aurora in our solar system, we can study it to better understand fundamental questions about how particles get accelerated or how plasma (i.e. ionized gas) shifts within a planet’s magnetosphere. In their recent paper, a group in the Astrophysical Sciences Department at Princeton, led by Dr. Jamey Szalay, investigated some of the finer details of how Jupiter’s aurora forms. Specifically, Szalay et al.’s analysis of data collected by the spaceship Juno demonstrates the importance of potential fields (i.e. how a mass is affected by an energy field) in accelerating protons away from Jupiter’s poles in the vicinity of its main auroral emissions.
One unusual property of Jupiter’s magnetosphere is the total abundance of protons. Jupiter’s moon, Io, contributes to the majority of ions in Jupiter’s magnetosphere in the form of heavy ions like Sulfur and Oxygen. However, about 10% of the ions around Jupiter are protons that cannot come from Io. There are three possible hypotheses that could explain where the excess in protons come from: a) solar wind, b) Jupiter itself, or c) its moons. The solar wind primarily consists of protons, so it would be reasonable to think that it might be the origin of the excess protons. However, Jupiter’s magnetosphere diverts solar wind around the planet, like a rock pushing water in a river. Therefore, it is unlikely that solar wind is the source of protons, as it would be difficult for a significant portion of protons to leak through Jupiter’s magnetosphere. Jupiter’s moon Europa could contribute some ions; the atoms surrounding Europa interact with and disturb Jupiter’s surrounding plasma, but not enough to account for the full proton excess. While Jupiter’s other icy moons contain water (a possible source of H+ ions), it is not clear how these particles would migrate inward to populate the innermost regions of the magnetosphere with protons. Therefore, it has been theorized that the proton excess must originate from Jupiter itself.
Recently, data collected from spaceship Juno showed protons accelerating away from Jupiter near the poles, a phenomenon that could directly explain the abundance of protons in Jupiter’s magnetosphere. While various theories have pointed to possible mechanisms, Szalay et al. used this data collected by Juno to find experimental evidence to support the theory that a specific setup of electric fields is the main mechanism driving protons out of Jupiter’s poles. By understanding how the protons arose from Jupiter, they were able to account for the excess protons and explain where protons originate from in Jupiter’s magnetosphere.
Before discussing the finer details of these results, it is important to briefly explain how the data was collected from the Juno spaceship. The spaceship makes an oblong orbit around Jupiter, traveling at high speeds near the planet’s poles and at low speeds at the outer reaches of its orbit. Two of its instruments, the Jovian Auroral Distributions Experiment (JADE) and the Jupiter Energetic particle Detector Instrument (JEDI), measure the energy of charged particles and to what extent these particles are aligned with Jupiter’s magnetic field. In this way, Juno is perfectly positioned to understand the dynamics between the plasma surrounding Jupiter and its magnetosphere. By using Juno’s recordings of proton flow, Szalay et al. were able to piece together the mechanism underlying Jupiter’s “faucet” of protons.
As Juno crosses the polar region, near where the aurora appears, its measurements of proton flow provide key insights into the auroral outflow. Specifically, it showed that the energy of these proton beams roughly follow an inverted-V shape, a shape known as an energy well. One way to conceptualize energy wells is to imagine a valley between two mountains: objects will flow into the valley, following a gravitational gradient. While electrical gradients are not as easy to “see,” they function in much the same way, where protons are pulled towards the well. The protons that move out of Jupiter’s ionosphere flow down an electrical gradient, where the high potential is near Jupiter (think of it like the mountain) and the low potential is somewhere in space (think of that as the valley). This outflow and the associated fields are likely related and produced by Jupiter’s complex auroral interactions.
The electric potential is thought to arise from the tension between two forces: Jupiter’s fast spinning (centrifugal force) and its gravitational pull. These two forces work against each other, one pulling in, the other pushing outward, creating differences in the density of plasma along the magnetic field lines. The uneven distribution of ions results in electric potentials. This potential causes the protons to shoot away from Jupiter’s ionosphere along the magnetic field lines. It is hypothesized that this potential structure could also explain the presence of the protons in the first place. As the protons shoot outwards, Juno’s measurements reveal that there are fewer free electrons near the energy well. The negative charge of the electrons causes them to move opposite the protons within the potential field, a phenomenon that has also been observed on earth. Szalay et al.’s key finding is that proton beams inject protons to Jupiter’s magnetosphere.
Szalay et al.’s analysis of Juno’s data provides an explanation for the previously mysterious excess of protons present in Jupiter’s magnetosphere. The novel observation of the “inverted V” shape in the measures of proton energy across Jupiter’s poles provides evidence that the excess protons are scoped out by potential energy wells, which pull protons off Jupiter’s ionosphere. More broadly, Szalay et al.’s work provides observational evidence for a Jupiter-origin theory regarding the creation of proton beams. Additionally, Szalay et al.’s work thoroughly characterizes the observed proton beams, creating a foundation for future study of planetary interactions with their local plasma environments. Understanding the source of excess protons in Jupiter’s magnetosphere allows scientists to confirm hypotheses about Jupiter’s aurora and plasma dynamics in general. This is particularly relevant because potential energy gradients, such as the one found on Jupiter, have also been found on Earth.7 This connection between planets allows a discovery on one planet to lay the foundations for understanding the other. In this way, by studying the mechanisms underlying both planet’s auroral processes, it is possible to come up with general principles that guide astrophysical phenomena.
The original letter was published in Geophysical Research Letters on December 11, 2020. Please follow this link to view the full version (paywalled).
References
1 Greicius, T. (2015, April 7). The solar system and Beyond is Awash in Water. NASA. Retrieved February 4, 2022, from https://www.nasa.gov/jpl/the-solar-system-and-beyond-is-awash-in-water/
2 Hille, K. (2015, August 4). Jupiter's Great Red Spot: A swirling mystery. NASA. Retrieved February 4, 2022, from https://www.nasa.gov/feature/goddard/jupiter-s-great-red-spot-a-swirling-mystery
3 Bloom, L. B. (2021, June 29). 22 best places to see the Northern Lights in 2020. Forbes. Retrieved February 4, 2022, from https://www.forbes.com/sites/laurabegleybloom/2020/12/30/best-places-to-see-the-northern-lights-in-2020/?sh=46f4b13d77d7
4 Fox, K. (2021, January 29). Aurora: Illuminating the sun-earth connection. NASA. Retrieved February 4, 2022, from https://www.nasa.gov/aurora
5 Image Source: “Science Learning Hub – Pokapū Akoranga Pūtaiao, University of Waikato, www.sciencelearn.org.nz”
6 Szalay, J. R., Allegrini, F., Bagenal, F., Bolton, S. J., Clark, G., Connerney, J. E., Ebert, R. W., Ergun, R. E., Mauk, B., McComas, D. J., Valek, P., & Wilson, R. J. (2021). Proton outflow associated with Jupiter's Auroral Processes. Geophysical Research Letters, 48(1). https://doi.org/10.1029/2020gl091627
7 Ray, L. C., Su, Y.-J., Ergun, R. E., Delamere, P. A., & Bagenal, F. (2009). Current-voltage relation of a centrifugally confined plasma. Journal of Geophysical Research: Space Physics, 114(A4). https://doi.org/10.1029/2008ja013969